Safe, abundant, carbon-free energy—this is the promise of nuclear fusion technology.
But fusion, the complex atomic process that powers the sun, is not yet feasible outside research settings here on earth. University of Tennessee, Knoxville, researchers aim to change that.
“The realization of controlled thermonuclear fusion as an energy source would transform society,” said Zinkle Fellow and Assistant Professor of Nuclear Engineering Livia Casali. “Fusion’s benefits can address many of the most pressing challenges facing the world today, including climate change, energy security, and the need for sustainable development. Fusion research is a critical investment in our future.”
UT is making that investment. “UT is a recognized leader in materials behavior in extreme environments, such as the tremendous heat and pressure inside a fusion reactor,” said UT-ORNL Governor’s Chair in Computational Nuclear Engineering Brian Wirth, who will become head of the Department of Nuclear Engineering in the Tickle College of Engineering in July. “This is further strengthened within the nuclear engineering department, which is consistently ranked among top US programs. We currently have seven faculty with strong fusion-related research programs.”
- Brian Wirth in the Low Activation Materials Development and Analysis Lab
- David Donovan and a graduate student in the Plasma Exposure Lab
UT has committed to further expanding that expertise with funding from the UT–Oak Ridge Innovation Institute, which was established in 2021 to enhance the long partnership between UT and Oak Ridge National Laboratory.
UT-ORII funds will go toward recruiting and hiring five researchers at each institution, building capabilities to address the most pressing challenges to fusion technology feasibility by focusing on three research themes: magnets, materials, and modeling.
By cohesively building their capabilities, UT and ORNL can also attract large-scale game-changing funding for collaborative research. In a race to turn fusion’s promise into reality, the United States government recently increased funding for fusion energy research in the 2024 appropriations bill, and more than $6 billion in private equity has been committed to developing fusion technology. Tennessee Governor Bill Lee has committed to advancing the state’s national leadership in nuclear energy and driving continued investment in its nuclear industry.
“There is tremendous opportunity and need to increase nuclear power to help meet our country’s rising energy demand,” said Wirth. “Projections suggest growing industries and data centers will need substantially more energy in the near future.”
UT will continue to strengthen its role in building an East Tennessee research, education, and industry ecosystem that will help transform nuclear fusion into an economically viable and environmentally beneficial energy option for the US.
“The combination of existing strength, the unique partnership with ORNL, and the research capability being added through the UT-ORII investment makes this region a natural for expansion in fusion energy and technology,” Wirth said.
Nuclear science and engineering are central to a wide variety of important applications, from energy to national security, space travel, food and water safety, and medicine.
Earlier this spring, UT-ORII committed funds for building UT’s and ORNL’s capabilities in radiopharmaceutical therapies to develop the next advances in precision cancer medicine.
“Both nuclear energy and radiopharmaceuticals use nuclear reactors and radioactive materials to serve the public,” said Wes Hines, Postelle Professor, Chancellor’s Professor, and current head of the Department of Nuclear Engineering (who was named UT’s 2024 Macebearer). “With nuclear energy, every day is about trying to protect people and the environment while powering rewarding lifestyles. Nuclear medicine is about enabling people to enjoy that lifestyle by extending their lives and quality of life.”
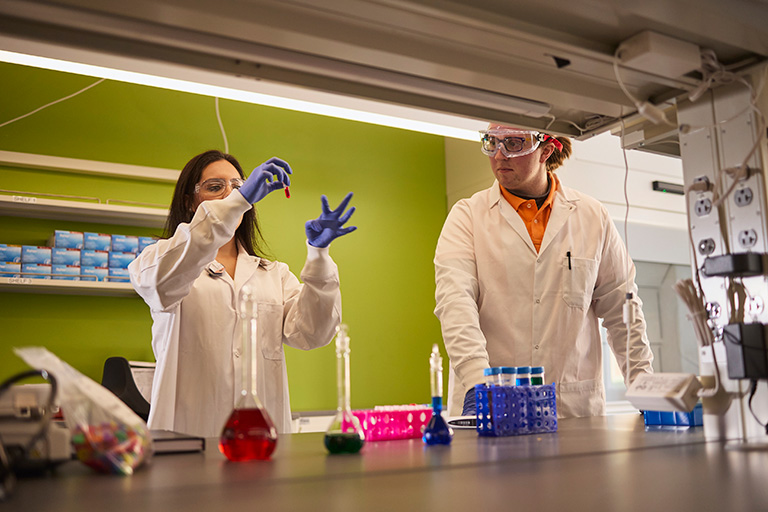
Ivis Chaple Gore with a graduate student
Nuclear engineering faculty are advancing both energy and medical applications. UT plans to hire additional faculty with radiopharmaceutical skill sets to work with existing faculty members such as Assistant Professor Ivis Chaple Gore. As a radiochemist, Chaple Gore focuses on radiopharmaceutical development, isotope production, and molecular imaging.
Understanding Magnet Performance
Magnets, Wirth said, “are at the heart of the promise of private fusion companies.” UT and ORNL research will complement private industry efforts to build fusion reactors by advancing the fundamental science of high-temperature superconducting (HTS) magnet performance.
Fusion takes place only under immense pressure and heat, at over 100 million degrees Celsius. At those temperatures, the fuels—two forms of hydrogen called tritium and deuterium—become plasma. HTS magnets create a magnetic field that confines the plasma and moves it in specific ways within the reactor.
“The last 20 years have seen tremendous improvements in magnets that can operate in high temperatures—but for HTS magnets, that means somewhere between -190 to -250 degrees Celsius,” Wirth explained. “We don’t yet understand how the temperatures and high-energy particle radiation in the fusion reactor degrade the magnet’s ability.”
“We need to develop a better fundamental understanding of the tolerance of HTS magnet materials to internal defects,” Wirth added. “That will be a critical part of assessing design and operating limits for these magnets.”
Today’s nuclear power plants use fission, the process of splitting apart atoms like uranium or plutonium. In contrast, fusion combines atoms, and fusion reactors typically use forms of hydrogen as fuels. Both processes generate carbon-free energy.
“Fission and fusion technologies are complementary,” Wirth said. “Ultimately, I think we need as much fission and fusion as we can get along with a host of renewable technologies like solar and wind.”
“While viable fusion power plants are still further off, advanced nuclear fission plants are already on the horizon,” Hines explained. “Especially with small modular reactors (SMRs) that can be built more quickly and cost-effectively than conventional plants. First, we’ll see SMRs using water as the coolant. Later, SMRs will use coolants like molten salt or sodium. They’ll operate at higher temperatures, benefiting companies that need high-temperature process heat.”
“Current power plants are extremely safe,” Hines said. “Our challenge is to maintain that safety record while making them more and more economically efficient.”
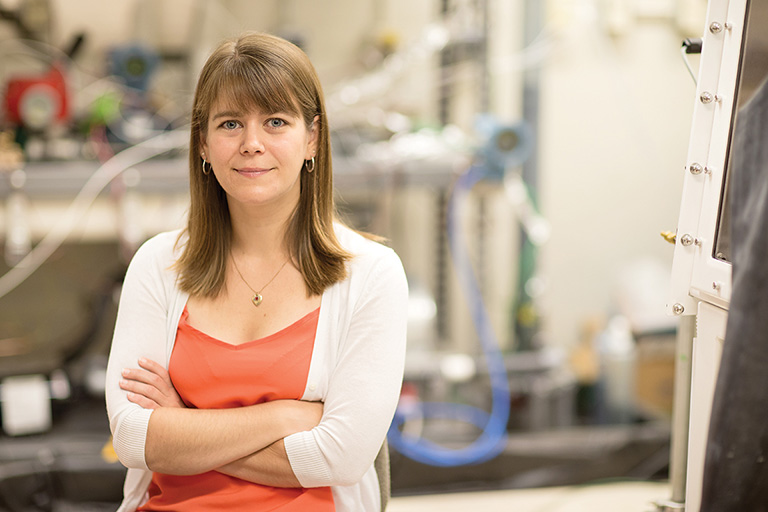
Jamie Coble
For example, Southern Company Faculty Fellow, Associate Professor, and Assistant Head for Undergraduate Studies and Service Jamie Coble is using advanced instrumentation and control techniques to better understand the condition of key equipment and overall plant performance. Her work informs optimized operations and maintenance strategies that contribute to safety, reliability, and economic competitiveness for current and future plants.
Improving Materials Made for Extremes
The materials research theme leverages UT’s and ORNL’s unique world-leading capabilities and facilities, including ORNL’s Manufacturing Demonstration Facility and High Flux Isotope Reactor as well as UT’s Tennessee Ion Beam Materials Laboratory.
- Several graduate students work in the Ion Beam Materials Lab
“We’re interested in developing advanced materials that provide sustained performance under high heat flux, high neutron flux, and corrosive coolants,” said Wirth.
“We have the capabilities to iteratively design, manufacture, and test fabricated composite structural component prototypes for fusion reactors,” he said. Research going forward will focus on components in three spatial regions of the fusion reactor: where material surfaces interface with plasma, where structural materials interface with coolant channels, and where the bulk of structural materials are subject to intense neutron activity.
“Testing the response of these material composites in the TIBML will allow us to capture the response to high-energy particle irradiation, and we’ll also test them in a high heat-flux testing facility,” Wirth said. “This data will then help us iteratively alter the materials to improve designs.”
TIBML Director and Associate Professor of Nuclear Engineering Khalid Hattar explained the lab’s role further: “We can explore coupled environments using laser heating, ion beam irradiation, helium implantation, and nanoscale mechanical testing. We will explore the thermal, irradiation, and magnet environments all at one time,” he said.
Using small-scale material samples, researchers in the TIBML can rapidly yet systematically test and screen new advanced alloy compositions for plasma-facing applications.
For more than a decade, the Tennessee Ion Beam Materials Laboratory has housed an ion accelerator that enables materials testing. A few years from now, it will boast four ion sources.
“We are in the middle of a major evolution,” said Hattar, “to a multiapplication multienergy accelerator facility to make far-from-equilibrium materials and understand materials evolution in controlled coupled extreme environments.”
“The multimillion-dollar investment in this core facility will enable us to replicate environmental conditions you would see in a fusion reactor,” said Hines.
The first step was procuring a custom scanning transmission electron microscope. “With this, we can see the defects that occur as ions hit the test materials,” Hines said.
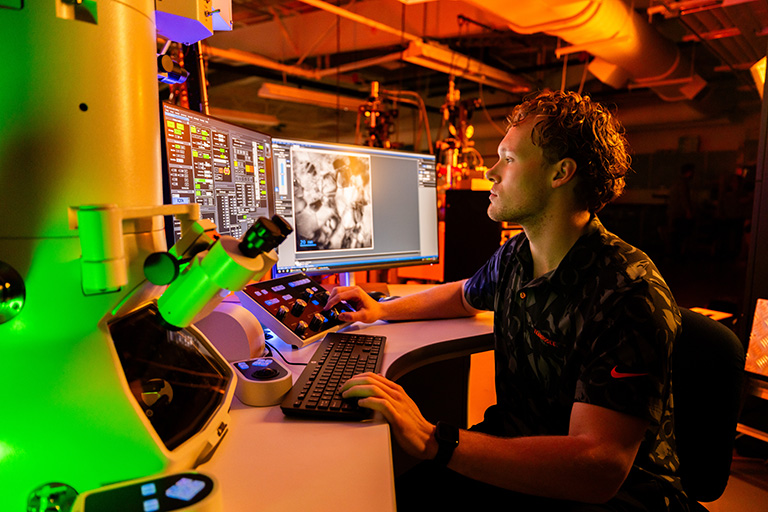
A graduate student uses the scanning transmission electron microscope at IBML
“The upgrades,” said Hattar, “will be able to advance our ability to understand materials evolution with nanometer resolution in very controlled extreme environments.” JEOL, the microscope manufacturer, has named the facility a center for excellence for in situ transmission electron microscope experiments.
Modeling a More Complete Picture
Even with facilities as advanced as TIBML, no single research facility can currently replicate every factor in the actual fusion power plant environment. That limitation makes modeling extremely important. UT and ORNL researchers have been tasked with developing and leading modeling efforts related to both magnets and materials.
“Rapid advancements have been made in various multiscale models to understand plasma–materials interactions,” Hattar said, “but the models we develop must still be validated.”
Materials and magnets experimentation will inform, validate, and refine models to effectively predict component behavior when exposed to a single environment. Researchers will then use that ability to predict material and magnet degradation in the setting of a prototype fusion power plant.
TIBML will serve an important role in modeling research, too: “The experiments we hope to do will validate or refine the multiscale models that UT and ORNL teams will develop,” Hattar said.
UT and ORNL researchers will also be bringing the power of machine learning to fusion modeling. “Machine learning and AI can sift through extensive datasets to uncover trends and predictive understanding,” said Wirth. “They can help us extrapolate beyond current computational and experimental datasets,” he added. That capability will be critical for modeling the environment of a prototype power plant.
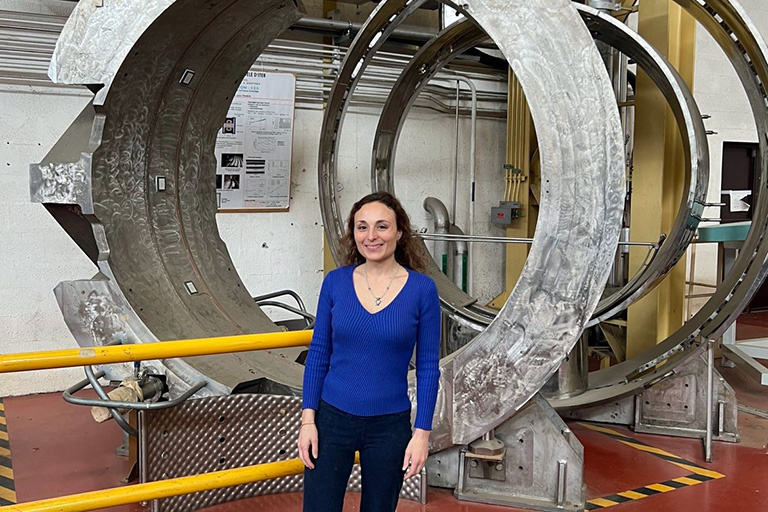
Livia Casali
Zinkle Fellow and Assistant Professor of Nuclear Engineering Livia Casali focuses on the interactions between the plasma’s hot core and its relatively cooler edges, where it meets structural components. “Because the core and the edge are governed by different physics,” she explained, “understanding how these regions interact and can be integrated represents an extraordinary challenge encompassing a wide range of spatial scales and a broad energy and temperature range.”
Tackling that challenge requires Casali and her team to use experimental data and develop state-of-the-art computational modeling. Ultimately, the synergy between the two can help develop operational scenarios for fusion power plants.
“My graduate students are involved in each stage of research,” she said. “Everyone in my group works on both experiments and modeling to understand how they relate to each other.”
Casali and her students travel the world to advance the science of fusion, collaborating with experimental and theory teams at facilities including Lawrence Livermore National Laboratory in California, the Max Planck Institute for Plasma Physics in Germany, and the International Thermonuclear Experimental Reactor under construction in France.
One of their main projects is connected with the DIII-D National Fusion Facility in San Diego. “Last year, I led the first-ever high-radiation experiments in the revolutionary negative triangularity tokamak configuration as part of my Department of Energy Early Career Award and a large international collaboration,” Casali said. “This tokamak configuration may be a game changer to improve exhaust handling and particle confinement to make future fusion energy plants more efficient and cost-effective.”
“Together with my students, we have analyzed experimental data and performed first-of-its-kind core-edge modeling,” she said. Her team has been invited to present their initial results at the International Conference on Plasma Surface Interaction next month in France.
Casali is also pursuing projects to improve codes used to simulate the different aspects of the fusion environment and unify them. “One important project includes developing a new code framework that can deliver, for the first time, self-consistent transport of the plasma from the plasma-facing component all the way to the plasma core, including impurity transport,” she said. This work is funded under her DOE Early Career award. “Another project we are working on is the coupling of fluid edge codes with edge turbulence codes.” That work is funded by a DOE Scientific Discovery through Advanced Computing award.
Under yet another DOE award, she is involved in experiments and modeling at the MAST Upgrade, in the UK—the first tokamak to feature the Super-X divertor, an innovative exhaust system designed for improved component durability.
Preparing Tennessee for the Future
UT is building its fusion capabilities at the right time to align not only with private equity and federal funding priorities but also with state economic development efforts.
Governor Lee created the Tennessee Nuclear Energy Advisory Council in 2023 to investigate and advise on strategic ways to support the state’s nuclear industry. Hines has served as co-chair on the council’s Education/Workforce Work Group to develop plans for how the state can fill the wide variety of potential workforce needs for technology companies and their suppliers.
From TVA to small startups, more than 150 nuclear-related companies already call the Knoxville–Oak Ridge corridor home. Dozens more fusion energy and technology companies could site their headquarters or testing facilities in East Tennessee over the next decade.
UT students will be in the right place at the right time. Wirth anticipates new opportunities for graduate student projects and training, and eventually new undergraduate research opportunities and internships as well.
“Ultimately, fusion is the ideal energy source,” said Wirth. “It’s going to take a tremendous amount of research and technology development to make fusion economically attractive to add to the grid. We’re in a great environment—here in Tennessee and right here at UT—to make that happen.”
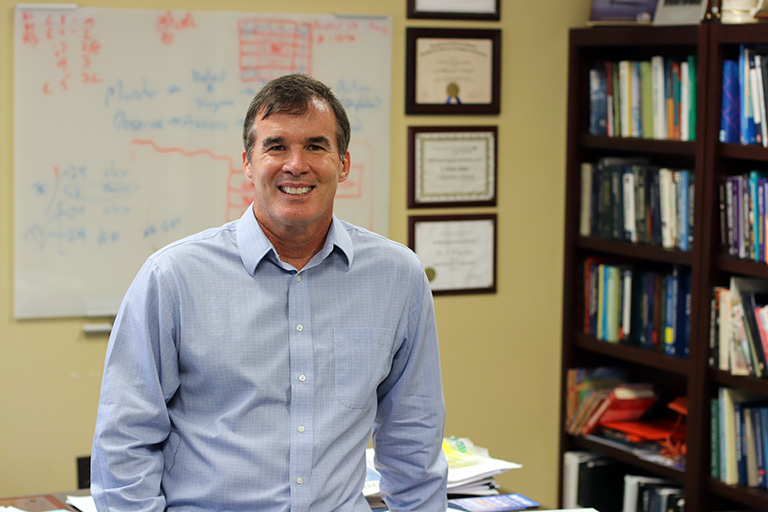
Wes Hines
“At UT, we want to be the pipeline for the engineers who will build, operate, manage, and maintain the nuclear plants of the future,” said Hines.
UT is in the right geographic position to do that. “The fusion company Type One Energy has announced plans to build their Infinity One stellarator prototype at the TVA Bull Run Plant and establish headquarters in Knoxville. Likewise, leading SMR companies are moving their headquarters to Knoxville. TVA is exploring building the very first SMR in the US that will produce power for the grid,” Hines said. “UT values our corporate partnerships, and we will continue growing these relationships.”
To that end, the university’s new Zeanah Engineering Complex includes a state-of-the-art facility where researchers and students can simulate operations of a nuclear power plant.
“Our partner Kairos Power will be putting their simulator in our facility,” Hines said. “We’ll use it for research purposes, to better understand how molten salt reactors operate, and to train our students—their future employees.”
“We’re also working with Kairos Power to develop training materials to help students and their current workforce get licensed by the Nuclear Regulatory Commission, which is considered the gold standard of nuclear safety for the world,” Hines said.